Respiratory Muscle Training
As expected, the loss of inspiratory muscle function is related to the level of injury as illustrated in Figure 1. Dyspnea, defined as a subjective report of breathlessness or shortness of breath, is common in people with SCI and is greatest in people with tetraplegia (Ayas et al. 1999). Approximately two-thirds of the prevalence of dyspnea in this group is attributed to the inspiratory muscle loss (Spungen et al. 1997). Improved inspiratory muscle strength and endurance could potentially improve cough and maximal exercise ventilation in addition to decreasing dyspnea. The inspiratory muscles can be trained like the limb muscles with inexpensive devices that increase the resistive or threshold inspiratory load on the inspiratory muscles (Reid et al. 2010). Table 10 outlines common measures that are indicative of respiratory muscle strength and endurance. In neuromuscular disorders like SCI, maximal lung volumes that measure IC also can reflect increased inspiratory muscle strength.
Evidence showing decreased dyspnea and improved strength and endurance after inspiratory muscle training (IMT) is well documented in healthy people (Karsten et al. 2018) people with other health conditions such as COPD (Reid et al. 2010; Geddes et al. 2005).
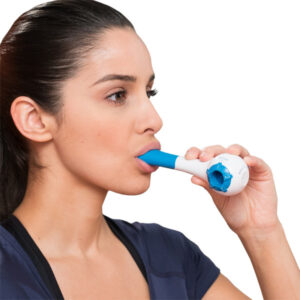
Figure 4. The Breather® Respiratory Muscle Trainer
Commercially available hand-held devices can be used for IMT. The four main types of devices are the resistive, threshold, isocapnic hyperpnea (IH), and incentive spirometers (Figure 5) (see Reid et al. 2010 for details of these training techniques).
- Threshold trainer: These devices, first used in patients with SCI by Ehrlich et al. 1999, have a one-way valve that closes during inspiration so that the person must breathe against a load; and the one-way valve opens during expiration such that no load is imposed during the expiratory phase of respiration.
- The resistive trainer imposes a load through a small diameter hole whereas the threshold trainer imposes a load via a spring-loaded valve. Regarding resistive trainers, also noteworthy for its widespread use, is the POWERbreathe However, as the authors of this chapter are aware, the only study using this model in participants with SCI showing the use of a hand-held electronic device (POWERbreathe KH1 device) was McDonald and Stiller (2019).
- IH imposes loading in a very different manner. The participant targets a prescribed ventilation level that requires higher inspiratory and expiratory flows. A bag attached to the device is adjusted to match the amount of rebreathing to maintain isocapnea i.e., a normal end-tidal CO2 level.
Figure 5. Inspiratory Muscle Trainers
Threshold trainer: Has an adjustable spring-loaded valve that imposes the inspiratory load. The inspiratory load can be increased by winding the spring more tightly. Advantage of this trainer is that the same load is imposed on the inspiratory muscles regardless of breathing pattern.
Threshold and P-Flex trainers available from Respironics HealthScan Inc., 41 Canfield Rd., Cedar Grove, NJ7, 0009-1201. 1-800-962-1266.
Resistive trainer: Has holes of different diameters. The inspiratory load can be increased by setting the dial to holes of lesser diameter. Disadvantage of this trainer is that the person can reduce the inspiratory load by breathing more slowly. If this device is used for training, a target must be used. Various targets have been designed that set a breathing rate (flow and/or inspiratory pressure) for the person.
Resistive trainer, POWERbreathe “PLUS IMT” device: Consists of an IMT device comprising a mouthpiece, a main body, and a regulator which, by means of a valve, allows controlling the air passage resistance, thus allowing the inspiratory muscles to be trained (González-Montesinos et al. 2012).
POWERbreathe “PLUS IMT” and POWERbreathe KH1 devices are available from POWERbreathe International Ltd., Northfield Road, Southam, Warwickshire, CV47 0FG, England, UK.
Resistive trainer, POWERbreathe KH1 device: Hand-held electronic device which provides a variable flow resistive load via an electronically controlled valve, with loading maintained at the same relative intensity throughout the breath (Charususin et al. 2013). This enables practitioners to quantify the inspiratory load during IMT (Langer et al. 2013).
Isocapneic Hypernea Trainer: Has a rebreathing bag that can be adjusted to ensure that the person’s CO2 level is maintained within a physiologic range. A target is provided for the person to increase the level of ventilation to a training intensity. This device enables training at low loads but much higher inspiratory and expiratory flow such that the inspiratory and expiratory muscles training at higher speeds of contraction. In contrast, the threshold and resistive trainers, place high loads while the speed of contraction is relatively low.
SpiroTiger™ trainer available from FaCT Canada Consulting Ltd. 1215 Cariboo Hwy N Quesnel, BC V2J 2Y3 Canada1-877-322-8348
Incentive spirometry (Coach 2® device, MediMark): An example of training was described by Shin et al. (2019), patients breathed through the mouthpiece slowly and as deeply as possible until the yellow piston indicator had reached the outlined area, to hold the breath for at least 5 s, and exhale slowly subsequently.
Portex® Coach 2® Incentive Spirometer available from 3Z DENTAL SUPPLIES. 100 Leek Crescent, Suite 5, Richmond Hill, ON, Canada L4B 3E6.
More recently, expiratory muscle training (EMT), has been added to IMT in patients with SCI as shown in some studies (Boswell-Ruys et al. 2020; Kader 2018; Kim et al. 2017b; Legg Ditterline et al. 2018; Gee et al. 2019) using complex devices (see examples of Expiratory Muscle Trainers in Figure 6).
Figure 6. Expiratory Muscle Trainers
PowerLung Trainer (PowerLung®): Devices which have spring-loaded inspiratory and expiratory valves by which the pressure threshold could be adjusted as Gee et al. (2019) showed in their study.
PowerLung® Trainer model available from PowerLung Inc. 1918 Triway Ln Houston, TX 77043 USA
Threshold PEP and IMT (Respironics): Devices which compound threshold positive expiratory pressure and inspiratory muscle trainer which could be assembled together in order to provide resistance to the expiration and to inspiration as showed Aslan et al. 2016; Legg Ditterline et al. 2018; Kader 2018).
Threshold PEP and IMT available from Philips Respironics. 1010 Murry Ridge Lane, Murrysville, PA 15668, USA
Respiratory training with bi-directional resistance will be considered RMT in this section (table 10).
Discussion
Two meta-analyses (Tamplin & Berlowitz 2014, N = 212; Wang et al. 2020, N = 448) demonstrated RMT improved pulmonary strength and respiratory parameters in people with SCI. Participants showed a range of improvements in VC, MIP, MEP, and MVV. Similarly, Lemos et al. (2020) found improvements in pulmonary function and respiratory muscle strength/endurance, but showed no effect on improving cardiorespiratory fitness (i.e., VO2max). Due to major variability across studies, they also could not establish which RMT type and protocol should be used to maximize benefits in athletes and non-athletes with SCI.
Studies have suggested that inspiratory resistive training (Mueller et al. 2012 and 2013; Soumyashree & Kaur 2020; Postma et al. 2014; Raab et al. 2019; West et al. 2014), respiratory muscular training (Boswell-Ruys et al. 2020), and normocapneic hyperpnea training (Van Houtte et al. 2008) significantly increases inspiratory muscle strength. Raab et al. 2019 and Soumyashree & Kaur 2020) found that IMT could increase expiratory muscle strength. Other researchers found similar success in improving pulmonary function (as measured by FVC, FEV1, PEF, PEFR, MVV and/or FEV1/FEV ratio) using IMT, RMT alone and when pairing RMT with an additional abdominal drawing-in maneuver (Aslan et al. 2016; Kader 2018; Shin et al. 2019; Kim et al. 2017b; West et al. 2014). Two RCTs provide level 1a evidence that RMT (Boswell-Ruys et al. 2020) and IMT (Soumyashree & Kaur 2020) improve functionality and exercise capacity as measured by 12 minute wheel chair aerobic test (12-MWAT), multistage fitness test (MSFT), and the six minutes push test (6-MPT).
Some studies combined various breathing techniques as part of a complex rehabilitation protocol. Chen et al. (2016) showed that patients receiving pulmonary rehabilitation, including breath training (lip breathing and abdominal breathing) and strength training (upper limb training such as arm crank cycle training) improved pulmonary function and life-quality during the 12 months of training in participants with SCI, compared to patients of the control group. Meanwhile, Shin et al. (2019) showed improvement in the absolute values of FVC, and PCF in patients who received RMT and care consisting of glossopharyngeal breathing (GPB) exercise, IMT using incentive spirometry, and air stacking exercises with a resuscitation bag for 4 weeks. The RCT published by Tamplin et al. (2013) showed that group singing exercises significantly improve phonation, and projected speech intensity; and Zhang et al. (2021) indicated that oral motor RMT and vocal intonation therapy improve IC, FEV1, maximal mid-expiratory flow rate, FEV1/FVC and SGRQ.
In addition, some studies have shown that RMT has the potential to dramatically reduce respiratory infections (RI) (Boswell-Ruys et al. 2020; Van Houtte et al. 2008).
Regarding parameters of training, great variability in dosage, repetitions, series, weekly frequency, and duration of training exists. Majority of studies reported that intensity of inspiratory and/or expiratory training was set at baseline with a range between 20% to 60% of MEP and MIP (Boswell-Ruys et al. 2020, Gee et al. 2019; Legg Ditterline et al. 2018; Postma et al. 2014; Raab et al. 2019; Soumyashree & Kaur 2020; Kader 2018; West et al. 2014). Studies described a progressive increase of the load (usually a 10% increase weekly or when RPE stabilizes or decreases) with a maximum load of 70 – 90% MIP and/or MEP at baseline; while one study reported that load increased weekly regarding MIP and MEP values were assessed weekly (60% MIP or MEP of last week) (Legg Ditterline et al. 2018).
Some previous studies could not be included in meta-analyses because of differences in training techniques or protocol, heterogeneity of participant characteristics, and/or differing measurement of outcomes. brooks
Future research to more accurately determine a treatment effect of IMT, EMT, and RMT after SCI should use: 1) larger samples; 2) outcome measures of inspiratory – expiratory muscle strength and endurance, dyspnea, QOL, and daily function; 3) optimal training techniques of threshold loading, targeted resistive devices, or normocapnic hyperpnea; 4) a comparison of the effectiveness of IMT, EMT, or RMT relative to or as an adjunct to other rehabilitation interventions. Of equal importance, overly aggressive prescriptions of IMT can fatigue and injure the inspiratory muscles, increasing the person’s predisposition to respiratory compromise. Reid et al. (2010) outlines parameters to monitor during IMT in order to avoid untoward responses such as muscle fatigue and hypercapnia. Parameters include: intensity of load, mode of load, duration, frequency and length of training to ensure adequate training protocol; blood pressure, heart rate, respiratory rate, other signs and symptoms of respiratory distress or inability to tolerate exercise load as signs of exercise intolerance; discoordinate chest wall movement, excessive dyspnea during training, long lasting complaints of fatigue after training sessions to avoid inspiratory muscle fatigue; signs of delayed-onset muscle soreness, reduced strength and endurance to avoid muscle injury; and end-tidal CO2, SpO2 and signs of headache, confusion to avoid hypercapnea (Reid et al. 2010). Van Houtte et al. (2008) provided 48 hours rest after their participants were unable to tolerate an overly intense workload.
For IMT to improve ventilation, decrease dyspnea, and to improve daily function after SCI, parameters to optimize IMT are currently only available for people with other respiratory conditions. For people with COPD, the optimal IMT protocol should use threshold or targeted resistive trainers:
- At an intensity of 30-70% of MIP,
- For a duration up to 30 min per session, performed continuously or in intervals, 4-6 days/week and be continued indefinitely (Geddes et al. 2006).
- Progression of intensity (MIP) should not exceed 5% per week.
Conclusion
There is level 1 evidence (from two RCTs: Boswell-Ruys et al. 2020; Kim et al. 2017b), level 2 evidence (from one prospective controlled trial: Kader 2018), level 3 evidence (from one case-control study: Aslan et al. 2016) and level 4 evidence (from two pre – post studies: Legg Ditterline et al. 2018; Gee et al. 2019) that RMT (IMT + EMT) as an intervention will improve inspiratory and expiratory muscle strength, pulmonary function and functionality and exercise capacity in people with SCI.
There is level 1 evidence (from three RCTs: Soumyashree & Kaur 2020; Van Houtte et al. 2008; Postma et al. 2014), level 2 evidence (from three RCTs: Mueller et al. 2012/2013; Loveridge et al. 1989; West et al. 2014), level 3 evidence (from one retrospective study: Raab et al. 2019), and level 4 evidence (from several pre-post and case studies) to support IMT as an intervention that will improve inspiratory and expiratory muscle strength, pulmonary function, functionality and might decrease dyspnea and RI in people with SCI.
There is level 1 evidence (from one RCT: Kim et al. 2017b) that the performance of RMT combined with the abdominal drawing-in maneuver improves more in FVC and FEV1 than RMT alone in patients with chronic SCI.
There is level 1 evidence (from two RCTs: Tamplin et al. 2013; Zhang et al. 2021) that music and vocal intonation rehabilitation (e.g., group singing exercises, oral motor RMT, and vocal intonation therapy) improves phonation, projected speech intensity, IC, FEV1, maximal mid-expiratory flow rate, FEV1/FVC, and SGRQ in patients with SCI.
There is level 2 evidence (from one RCT: Chen et al. 2016), level 3 evidence (from one case control study: Shin et al. 2019), and level 4 evidence (from two pre – post studies: Leathem et al. 2021; Shanmuga Priya & Kalpana 2018) that different combinations of breathing training exercises, and general body exercises, improve pulmonary function, functionality and QOL in patients with SCI.
There is level 4 evidence (from one pre – post study: Zhang et al. 2016) that functional magnetic stimulation (FMS) conditioning is safe and effective to improve the inspiratory and expiratory function of patients with SCI.